Understanding CRISPR: A Revolution in Gene Editing
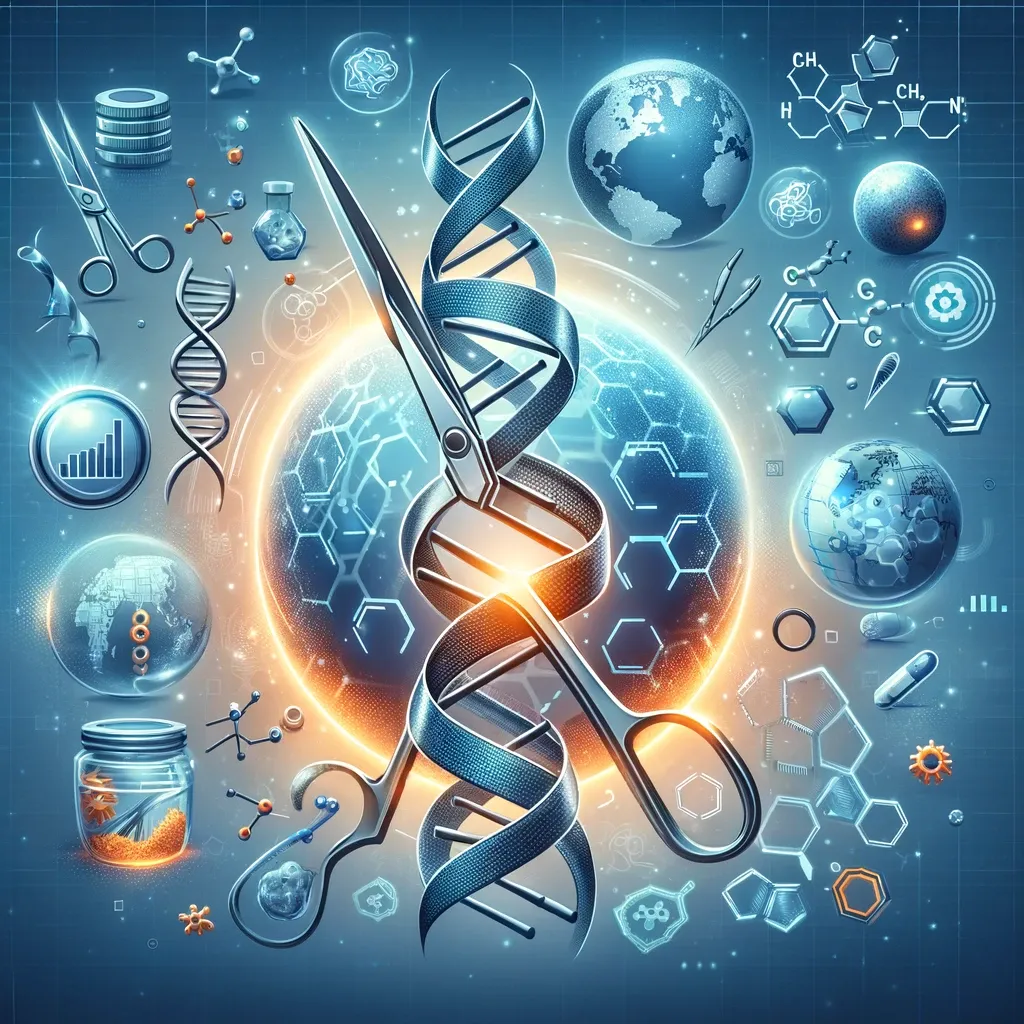
CRISPR, standing for Clustered Regularly Interspaced Short Palindromic Repeats, represents a significant leap in gene editing technology. Initially discovered as a part of the bacterial immune system, it has been repurposed as a powerful tool for modifying DNA. The core of CRISPR's functionality lies in its ability to act as molecular scissors, precisely cutting DNA sequences as directed by a customizable guide RNA (gRNA).
Key Components of CRISPR:
- CRISPR-associated (Cas) Nuclease: This enzyme is responsible for binding to and cutting DNA.
- Guide RNA Sequence (gRNA): Directs the Cas nuclease to the specific DNA target.
Originally found in bacterial immune systems, where it disabled invading viruses (bacteriophage) by cutting their DNA, CRISPR's DNA-cleaving capability has been harnessed for genome editing. Its discovery and development into a gene editing tool were significantly contributed to by Dr. Jennifer Doudna and Dr. Emmanuel Charpentier, who published a groundbreaking paper in 2012.
Why Is CRISPR Important?
CRISPR's importance cannot be overstated. It allows for precise genetic modifications in virtually any organism, surpassing older techniques in simplicity, affordability, and accuracy. Applications range from curing genetic diseases to creating crops that can withstand harsh conditions.
The History of CRISPR:
The journey to CRISPR's current status as a gene editing marvel was long. Its story involves several scientists, including Feng Zhang, George Church, and Virginijus Siksnys, who contributed to its understanding and application in various organisms. The first to adapt CRISPR-Cas9 for gene editing were Dr. Doudna and Dr. Charpentier, but its roots trace back to 1993 with Dr. Fransisco Mojica's identification of CRISPR sequences in bacterial genomes.
CRISPR Mechanism: How It Works
CRISPR functions as a part of adaptive immunity in bacteria and archaea. When these microbes are infected by a virus, the CRISPR system captures a fragment of the viral DNA, storing it as an immune memory. Upon reinfection, the CRISPR system uses this stored sequence to identify and destroy the virus.
The process involves:
- Viral DNA Fragment Capture: Cas nuclease snips off a piece of viral DNA.
- Immune Memory: The viral fragment is stored in the bacterial genome.
- Target Recognition and Destruction: Upon reinfection, the bacterium recognizes the virus and destroys it using the CRISPR-Cas system.
The realization that this natural system could be engineered to target specific DNA sequences led to its adaptation as a gene editing tool. Researchers now can program the CRISPR system to target any DNA sequence in a genome, using a custom guide RNA.
CRISPR-Cas9 Gene Editing:
This process involves creating double-stranded breaks in DNA at specific sites and leveraging the cell's DNA repair mechanisms. The two primary pathways used for gene editing are:
- Non-Homologous End Joining (NHEJ): Often used to disable genes.
- Homology-Directed Repair (HDR): Used for inserting new genes or genetic material.
CRISPR Techniques and Applications:
CRISPR's versatility extends to various techniques like gene knockouts, gene knock-ins, and CRISPR activation (CRISPRa) and interference (CRISPRi). Each method offers unique capabilities:
- Gene Knockouts: Utilizing NHEJ, CRISPR can induce insertions and deletions (indels) at the target site, potentially causing frameshift mutations that render genes non-functional. This approach is vital for functional genomics, drug discovery, and disease modeling.
- Gene Knock-ins: Through HDR, CRISPR enables the insertion of new DNA sequences or entire genes at the target site. This technique is crucial for creating cell and gene therapies to correct mutations causing human diseases.
- CRISPRa and CRISPRi: Modified versions of CRISPR, these techniques regulate gene expression without altering the DNA sequence. CRISPRa upregulates gene expression, while CRISPRi downregulates it. They are achieved by fusing a catalytically dead Cas9 (dCas9) with transcriptional effectors to the target gene.
CRISPR's Broader Impact:
Since its adaptation for gene editing, CRISPR has seen rapid progress and diverse applications. Its use in clinical trials, like those for sickle cell disease, exemplifies its potential in therapeutic contexts. Moreover, CRISPR continues to evolve, with ongoing research enhancing its efficiency and expanding its capabilities.
Free CRISPR Consultation:
For those seeking to optimize their CRISPR experiments, free discovery calls and consultations with genome engineering experts are available. These sessions provide insights into achieving guaranteed editing results, tailored to individual research needs.
Conclusion:
CRISPR stands at the forefront of genetic engineering, offering unprecedented precision and versatility. Its journey from a bacterial immune mechanism to a groundbreaking gene-editing tool underscores the transformative power of scientific discovery and collaboration.
Comments ()